Yotube.com
Development of large-scale, polymer-based sensor foils employing purely optical measurement principles
PlanOS aims to develop a new generation of entirely polymer-based optical foils on which large-area arrays of optical sensors are integrated. Using light to measure quantities such as temperature, strain or concentrations of chemical compounds, the PlanOS foils combine micro-optical components with large-area fabrication technology. Our vision is to provide sensor technology on a large-area, 100 μm thick, flexible polymer foil manufactured on an industrial scale. Manyfold applications are possible: from aviation industry to packaging as well as structural health monitoring and molecular analytics in life sciences.
Our chemists develop tailor-made materials for fabrication of optical waveguides and substrates. Physicists and engineers build light sources and detectors, and improve existing sensor technology such as arrayed-waveguide and fiber Bragg gratings. Other scientists develop new concepts for processing light in novel sensor networks. In addition, engineers work on the realization of a cost- and resource-efficient mass production.
PlanOS is a Collaborative Research Centre funded by the German Research Foundation (DFG). Project partners are the Leibniz Universität Hannover, the Albert-Ludwigs-Universität Freiburg, the Laser Zentrum Hannover e.V., the Technische Universität Clausthal, and the Technische Universität Braunschweig.
Supporting Institutions
-
Institute for Microsystems Technology
Albert-Ludwigs-Universität Freiburg
-
Institute for Energy Research and Physical Technologies
Technischen Universität Clausthal
-
Institute for High Frequency Technology
Technischen Universität Braunschweig
Projects in PlanOS
Project Block A
-
A01 - Polymer-Hybrid Materials
Supervisor: Prof. Rühe
In this project we will develop methods for the fabrication of thermally stable micro- and nanostructures in polymer foils for large-area micro-optic applications. The concept is based on a combination of chemical cross-linking of pre-polymers which contain thermally reactive groups, with methods of micro- and nanoimprinting. One-component systems of foil-forming copolymers will be used, which crosslink and preserve the molded structure during the exposure to higher temperatures as they occur during the embossing process.
In the proposed concept thermally activated CH insertion reactions cause the cross-linking processes. As a first example for groups, which induce the cross-linking process, (substituted) sulfonylazids are to be incorporated into the polymers.
The network formation renders the foils insoluble and, hence, stable against permanent deformation. Even a rounding of the structure, as it often appears during demolding, will be strongly reduced or even avoided. Depending on the cross-linking density and the type of the polymer used, optical foils with customized mechanical flexibility can be obtained.
To be able to adjust the optical properties of the foils, tailor-made composite systems consisting of the basic polymer components and inorganic nanoparticles will be developed. Mainly approaches based on sol-gel-processes will be investigated.
The influence of the composition of the polymers or the composites on the optical properties and thermal and mechanical stability of embossed micro-structured foils will be determined.
-
A03 - Polymeroptical Sources and Sinks
Supervisor: Prof. Kowalsky
In project A03 "Polymer Optical Sources and Drains" organic light emitting diodes and organic photo detectors are investigated in combination with optical waveguides. The goal of the project is to integrate organic light sources and detectors into polymer waveguides to get a completely organic based system. The challenge in this approach lies in the efficient coupling of light between those devices and the waveguide.
Suitable device designs and coupling structures have to be examined and evaluated for use in the final setup. The IHF has extensive knowledge in the field of OLEDs and organic solar cells which will be used as light sources and detectors respectively.
Figure 1 shows a system overview with OLED as optical source and OPD as optical sink. A polymer waveguide can be seen in Figure 2.
-
A04 - Laser-active waveguides based on nanoparticle-doped polymers
Supervisor: Prof. Chichkov
In this project, polymer-based optical elements will be developed, which will be used later as laser-active optical components (Figure 1). The major advantage of polymer-based materials, compared to glass matrices, is the ease in material processing, combined with the possibility of three-dimensional shaping, for instance by injection molding, embossing or laser lithographic techniques. Based on their general acceptance and usage in optical applications, in this project, Poly(methyl methacrylate)-based composites will be primarily in focus.
Optical active properties will be achieved through the homogenous embedding of various nanoparticles, including glass, crystalline, and rare-earth-based materials into the polymer matrix (later referred as nanocomposite). The required homogenous doping of polymers with nanoparticles will be achieved using pulsed laser ablation in liquids. This physical synthesis method allows the direct and controlled generation of nearly any kind of nanoparticles in various liquid environments. In order to successfully realize optical applications with these nanocomposites, nanoparticle agglomeration in the host polymer matrix must be thoroughly hindered.
For this purpose, in the Laser Zentrum Hannover e.V., a liquid-based material compounding technique has been developed.
As optical active nanomaterials, nanoparticles based on lanthanides (rare earth metals) are of particular importance, as these materials already found application in laser crystals. Therefore, in this project, lanthanide-based nanoparticles in diverse forms will be investigated. Firstly, nanoparticles will be generated from rare-earth doped glasses and crystals. Nanoparticles will be also produced directly from rare-earth metals and their oxides. Generated nanomaterials and nanocomposites will be studied by different analytical techniques to monitor their optical properties using UV-Vis absorption spectroscopy, scanning electron microscopy, and fluorescence spectroscopy (Figure 2). The use of different nanoparticles in nanocomposites will allow studying the influence of polymer matrix itself and the alteration of relevant optical properties such as fluorescence, scattering, and optical amplification.
For optical characterization of the nanocomposites, macroscopic model optical elements will be produced by injection molding. Moreover, within this project, active and passive waveguides will be fabricated using imprinting techniques.
-
A05 - Optodic Bonding and eutectic bonding of Silicon Photonics on Thin Flexible Interposers
Supervisor: Prof. Overmeyer and Prof. Maier
The long-term goal of our consortium SFB/TRR 123 is to produce optical functionalities directly within the polymer film. While this is being researched by project partners A03 and A04, we will also adopt silicon photonics. Hybrid solutions combining silicon photonics and polymer photonics will help solve a major part of our sensing applications. In particular, silicon photonics will mainly serve as a laser source and an analyzer of optical spectra within the film.
Mounting of chips on thin polymer films is the state of the art in the field of RFID tagging. Usually thermally curing adhesives are employed, which require long processing cycles or a temperature insensitive interposer. We believe that light driven bonding techniques are advantageous due to the absence of thermal loading. Optodic bonding is indeed of value for thermally sensitive substrates like low Tg polymers: e.g. PMMA, PET and PVC. It is our goal to develop an embedding solution for silicon photonics on thin polymer interposers with minimal thermal loading or even with complete absence of thermal loading.
In this part of the project, we follow two approaches to mechanically embed opto-electronic components, e.g. LEDs, onto a thin flexible interposer. One possibility is to use adhesives that cure with UV light. This method allows mechanical and electrical contact between the interposer and the opto-electronic component. In this approach, the UV light wave is transmitted through the interposer to the contact region between component and interposer. The second approach constitutes of the use of eutectic bonding where a laser source is used to transmit thermal energy to the contact region. In order to reduce thermal loading effects on the interposer, eutectic bonding happens at low temperatures in a localized and timely concise manner. We aim to achieve contact strength equal to the one achieved from classical eutectic bonding; 20 MPa. In order to protect the interposer, process temperatures are to be kept under 90°.
Our metrics of success for both approaches are mechanical strength, electrical resistivity at the contact region and optical transmission. In terms of optical functionality, while the adhesive is an optic medium, the eutectic bonding region is to be wrapped with an optically active underfill. Project partners, B01, investigate the use of inkjet printing to optically connect opto-electronics with light waveguides embedded within the interposer. Moreover, hot embossing of coupling structures within the flexible interposer will enable coupling of light between waveguides and opto-electronic components. This is being done by our project partners B04.
In order to transmit optical signals, LEDs, laser diodes and photo diodes with a wave length ranging from 400 nm to 1100 nm will be used. Figure 2 depicts the general set up that will be utilized to research optodical bonding.
While a UV light source is necessary for curing the adhesive, LED-UV lamps can be used (Figure 3), in which an LED-UV lamp is mounted under a transparent chuck within a flip chip bonding station (left) or the LED-UV lamps are installed on the top (right).
In terms of eutectic bonding, we will proceed by connecting 3D electro-optical components on a flexible interposer in two stages. In the first stage of our research, we will use a carrier based on a semiconductor material to help connect 3D components with the optical interposer. Here, the main focus is on the functionality of the 3D components without being constrained by the size of the connecting region. The reason behind this approach is to provide the partners in our consortium with light sources and sinks at an early stage of the project to be able to investigate the feasibility of polymer light waveguides for instance. In a second stage of our research, we will proceed by connecting the 3D components directly with the optical interposer. We will build on the utilization of carriers based on semiconductor materials. In this stage of the project, the carrier will serve as an electrical re-router.
In conclusion, the ultimate goal of both optodical bonding strategies is to deliver a reliable method for connecting LEDs, laser diodes and photo diodes to an optical thin flexible interposer.
Project Block B
-
B01 - Flexo- and Inkjet-Printing of Multimode-Waveguides
Supervisor: Prof. Overmeyer and Prof. Korvink
To guide light through a planar optronic system, one requires optical waveguides. While, typically, glass is used to build such devices, the research project PlanOS employs specifically tuned polymer materials.
All B-projects of PlanOS are investigating the creation of optical waveguides of different sizes. Project B01 is taking care of relatively broad waveguides with diameters comparable to the thickness of a human hair. Optical waveguides of this size are capable of carrying much light and therefore are the basis for optronic systems. For the manufacturing, two printing techniques are employed: For large area foils, flexographic printing, for smaller areas, inkjet printing.
Inkjet-Printing
When manufacturing optical waveguides, it is very important that the surface of the structures is of optical quality, which means extremely flat. Roughness has the effect that the light is not cofined into the waveguide, but refracted into its surroundings. Inkjet printing is technique where extremely small amounts (a few picoliters) of a liquid are deposited onto a surface droplet by droplet. After landing there, they have time to flow together to form a structure with a perfectly homogenuous surface, just like a raindrop on glass. Because of this mechanism, inkjet printing is very suitable for the creation of optical waveguides. As soon as the material on the substrate is at rest, the ink can be dried by heat or cured by UV-induced molecular cross-linking.
As a suitable viscosity is the only condition, a wide range of materials can be processed via inkjet printing, as long as they are available in liquid form. Additionally, only small amounts of a liquid are required to perform printing processes, which makes the work at experimental stage much easier, as often only small amounts of novel inks are available. However, the technique is based on the ejection of many single droplets after one another. Although this happens very fast (several thousand droplets per second), the process is relatively slow. For the manufacturing of large structures, another printing technique is more suitable.
Flexo-Printing
To allow the cost-efficient production of planar optronic systems with high throughput, flexographic printing is employed. The idea is to create the majority of the optical structures with this technique, and complement individual parts with inkjet printing after that. This way, a combination of both processes' advantages is possible.
Flexographic printing is a relief printing technique which is typically employed for the graphic printing of packaging. In this technique, a printing plate, which functions similarly as a stamp, is mounted onto a rolling drum. The elevated structures which represent the intended printing pattern are first covered with paint. Then, rolling this drum over the substrate leaves the pattern on it. In recent time, the option of printing electrical circuits was investigated. In this project, flexographic printing is employed to realize optical geometries.
Flexographic printing has the advantage of having a very high throughput (up to 15.000 sheets per hour) and consequently very low cost per sheet. Additionally, flexographic printing machines are available all over the world, so an established production process would feasible on an industrial level.
The disadvantage in comparison with inkjet printing is that structured printing plates are required for the process. This means that the variability of the process is lower, because for a change of the printing motif requires a new printing plate to be manufactured. Additionally, the required resolution for thin optical waveguides could not yet be achieved with current methods.
The research is focused on realization of optical waveguides, which have a low attenuation and fulfill the requirements of the partner projects. For this, suitable inks of liquid polymers which are cured via UV-light are selected and adapted. Additionally, it is investigated how a high resolution and the intended cross section of the waveguides can be realized.
-
B02 - Nanoimprint lithography of singlemode waveguides
Supervisor: Prof. Müller
The project objective is the fabrication of passive, quasi-monolithic and high precision optical polymer components over large active surface areas (70 cm²).
The central focus of the project besides the integration of the established techniques NIL and HE, remains on the development of a fabrication process that can compensate contraction and shrinkage of polymers, thermally induced or due to polymerization effects. Up to now both, contraction and shrinkage, have limited the combined structures with optical quality on large surface areas.
Structuring of polymers over large surface areas with high aspect ratios of up to 10:1 therefore generates an explicit challenge, while the optical quality of the structures has to be constantly controlled and maintained.
In order to fabricate adequate master- and NIL-moulds (see figure), combined processing sequences have to be developed in the scope of the project, e.g. ultra precision machining (UPM), electrical discharge machining (EDM), lithography & electroplating and casting with silicones (e.g. polydimethylsiloxane (PDMS)).
-
B03 - Polymer processing with femtosecond lasers
Supervisor: Prof. Morgner and Dr. Reinhardt
In the mid-90s it was first shown that materials can be shaped on a micrometer scale with the help of femtosecond lasers. Since then this technology has advanced at an enormous pace and nowadays material modifications on a nanometer scale are feasible. One interesting aspect is the modification of the refractive index in transparent materials. If the refractive index is increased compared to the surrounding material local optical waveguide structures can be fabricated. The writing of waveguides in glasses has been investigated intensively but transferring this technology to polymers turned out to be challenging, since the polymer matrix does not exhibit the same long term stability as glasses do. This leads to a decay of the waveguide structures up to the point where wave guiding is no longer possible. For that reason glass is often preferred as construction material. However - due to their mechanical properties - polymers hold potential for applications which cannot be implemented in glass. One could for example imagine waveguide structures being written into a foil and having this flexible material applied on a surface of any given shape.
Another way to manufacture waveguides in polymers is the two-photon polarization method (or short 2PP). This technique of direct laser writing was demonstrated in 1992 and was first used to create miscellaneous three-dimensional micro structures. For the 2PP-technology the beam of a short pulsed laser is focused into the volume of the polymer. Contrary to the previously described material modification; in this case the polymerization process of monomers or the crosslinking of polymers is ignited by two-photon absorption to create microstructures. With the development of adequate materials and laser systems this technique has been used to create three-dimensional photonic crystals since 1997. The nonlinearity of 2PP allows the fabrication of any computer generated 2D or 3D structure with a resolution even below 100 nm. Despite this promising potential up to now this technology has rarely been used for the fabrication of optical waveguides since no waveguides in the sub-micrometer range were required. Only in 2006 the first 2PP polymer waveguides were created on a thin metal film, which were used as waveguides for surface plasmons. For this purpose the dimensions of the waveguide need to be well defined below the 1 micron limit to prevent higher waveguide modes from developing. With 2PP a number of optical elements could be demonstrated in the micrometer range.
Future applications of these structures include – depending on the polymer – ultrafast optical or electro-optical switching elements, logical optical elements, tightly arranged sensors or compact polymer laser amplifiers. To realize such optically active waveguide structures the development of groundbreaking polymers with improved long term stability or novel optical properties is required as well as an all-purpose technology to flexibly and cost efficiently machine these materials. The fabrication of waveguides via femtosecond laser radiation offers multiple advantages compared to other technologies. On the one hand it is not limited to just one plain; with the help of computer controlled translation stages the material processing is possible along virtually arbitrary three-dimensional paths. On the other hand this technology is insensitive towards the environment since the material modification takes place within the bulk material. Therefore no clean room conditions are needed.
This sub-project works on the development and supply of a technology to fabricate high-quality 2D and 3D optical waveguides and waveguide systems in different polymers. The foundations for the proposed technologies are the two above mentioned nonlinear laser lithographic structuring methods via femtosecond lasers:
-
Three dimensional writing of waveguides in the volume of a polymer by material modification; analogous to waveguide writing in glass.
-
Three dimensional structuring of waveguides by two-photon polymerization.
Combined these two approaches enable the fabrication of waveguides in different material systems that could not be covered by just one. The focus will lie on three dimensional waveguides of Type I and Type II, where the wave guiding takes place either directly in the modified region (Type I) or in between multiple modified regions (Type II). Additionally the 2PP technique shall be used to fabricate two dimensional waveguides and grid structures on planar, flexible substrate. To achieve ideal functionality of the components computer simulations will be conducted that will help to find the appropriate waveguide geometries for different materials. 2PP can also be used for the making of three dimensional waveguides. Nano porous materials – like Polydimethoxysiloxan (PDMS) – can be soaked with monomers. As in direct refractive index modification in glasses and polymers the laser radiation can be focused deep into the volume of the nano porous matrix where monomers can be polymerized in three dimensions via 2PP.
The waveguides created with these methods are to be optically characterized and their properties regarding different coupling techniques shall be investigated. In cooperation with our project partners polymer microstructures are to be put into practice which can act as a beam splitter, wavelength division multiplexer (WDM), arrayed waveguide gratings (AWG), interferometers and planar sensor prototypes. The high precision and flexibility of the proposed methods enable a high value of compatibility and will render possible rapid prototyping of novel optical circuits on smallest areas.
-
-
B04 - Production of micro-optical components
Supervisor: Prof. Reithmeier and Dr. Rahlves
Within project B04, coupling structures and waveguides are designed and optimized regarding coupling efficiency and power extinction by carrying out numerical simulations. Since design and optical performance of a particular coupling structure strongly depends on its physical principle and, therefore, on the size of the microstructures, various coupling concepts have to be considered. The concepts are especially suited for optical devices, which are developed within other PlanOS-projects. Examples of coupling structures are shown in the figure above and are based on total internal reflection at a polymer–air boundary (left) or on light coupling using diffraction gratings (right). The scientific challenge when designing such coupling structures is the technical realization of the micro-optical structures, which are completely polymer based. A hot embossing process was chosen as most suited for this purpose. We intent to develop a new fabrication process based on hot embossing which is based on successive utilization of polymers with different glass temperatures without using UV curing polymers.
-
B05 - Functionalized surfaces and multilayers
Supervisor: Prof. Ristau
The goal of subproject B05 is the generation of flexible protection layers which can be applied to single components as well as to the entire foil.
A demanding challenge results from the conflict between high flexibility and high resistivity of the coatings with simultaneously sufficient adhesion to the polymer surfaces. Oxide coatings provide good mechanical protection, but they often suffer from fragility and delamination effects on polymers. A possible solution of this problem is a continuous transition from oxide to polymer within one layer [see fig. 1].
The manufacturing of these transitions can be realized by ion beam sputtering (IBS). This technique is well known for the production of highest quality complex oxide coatings in optical thin film technology. The basic principle of IBS comprises a sputtering of the coating material from a target by an inert gas ion beam and a subsequent deposition of this material on e.g. a glass or foil substrate [see fig. 2].
The geometrical degrees of freedom of IBS allow the use of zone targets with different adjacent materials. The continuous variation of the mixing ratio is achieved by positioning of the target material zones relative to the ion beam. While this process has been optimized for oxide materials since many years, ion beam sputtering of polymers is still at the beginning of its development.
Before this background, subproject B05 is dedicated to IBS process concepts for the deposition of different polymers. For example, transparent coatings with a refractive index of 1.38 could be produced from a polytetrafluorethylene (PTFE) target with IBS. Contrary to bulk PTFE, which exhibits a quasi-crystalline structure and scatters nearly 100 % of the incident light, the sputtered PTFE coatings are amorphous and nearly free of optical losses. In view of their low refractive indices, these coatings may also gain of interest for classical optical applications.
Sputtered PTFE reveals a relatively weak mechanical stability, and the mechanical stress of the coating is low. In order to improve the protective properties, sputtered PTFE was mixed with sputtered tantalum pentoxide for the first time by IBS within the subproject B05.
Starting from pure Ta2O5 coatings with a refractive index of 2 a decrease in refractive index down to 1.8 can be achieved by adding of PTFE without degradation of the optical quality. In addition the mechanical stress is reduced by a factor of four resulting in an improved compatibility to polymer substrates [see fig. 3].
-
B06 - Lamination via chemical bonding reactions
Supervisor: Prof. Rühe
This project aims at the development of a continuous manufacturing process to create micro structured multilayer systems for active and passive optical devices and systems in polymer foils.
The most important and established method to generate multilayer polymer foils is the connection of two foils by lamination processes that rely on thermal induced (partial) interdiffusion of polymer chains. Existing lamination processes reach their limits whenever a connection of incompatible, non-miscible polymers is desired. An example is the combination of poly(methyl methacrylate) (PMMA) and cyclic olefin copolymers (COC). Because of a compromising of the essential optical properties, an adaption of the materials regarding the lamination process is in most cases impossible.
This project aims at a new reactive lamination process that allows the connection of chemically diverse materials to attain multilayer foils by lamination. During this process, a compatibility layer of photo- or thermoreactive polymer material is spraycoated on one of two polymer foils that are to be connected. The connection of these materials shall be enabled by chemical interface reactions which can be thermally or photochemically induced. In order to attain this objective, new materials are to be developed that allow the connection of the different materials by recombination or insertion reactions via biradical or similar reactive intermediates (nitrenes). During the lamination process, a number of film layers shall be connected by covalent bonds. Protective layers or compatibility layers could be developed on the foil surface in analogy.
For the inclusion of specific optical devices (beam splitters, gratings), a combination of the lamination process and preceding or following processing via vacuum-thermoforming or nano-imprint technologies is to be investigated as well. Passive devices are to be developed first, followed by the design of active devices to attain an integrated development process.
The concept of approach could be proven by adding a thin photoreactive compatibility layer to a COC substrate, followed by lamination with incompatible PMMA foil. Fig. 2 illustrates a laminate, where the two foils were connected only in the compatibilized/coated area. Between coated and uncoated area, there is a white or opaque area. This area is a result of the crazing due to stress applied by a pull test, illustrating the stability of the laminated bond.
Project Block C
-
C01 - Micro- and nano-optical sensors
Supervisor: Prof. Zappe
The overall goal of the Collaborative Research Center “PlanOS” is the realization of a flexible polymeric foil, which serves as a distributed sensory surface. Optical sensors play a decisive role in this approach. They translate the measured quantities into optical signals, which are transferred through the foil to an evaluation unit.
This subproject focuses on the development and fabrication of polymer-based micro and nano-optical temperature sensors.
Measurement principle
Light is transferred through the foil by integrated waveguides. For temperature measurements an appropriate optical grating (so-called Bragg-grating) is integrated into the waveguides. This grating is designed, so that the entire incident wavelengths range is transmitted, except one wavelength, which is reflected back. Heating induces expansion of the polymeric material and of the Bragg-grating, thus changing the back reflected wavelength. The corresponding temperature can be calculated from the measurement of this shift.
Tasks and challenges
Polymeric materials are especially well suited for the described sensory application. Their high thermal expansion results in a high sensitivity and resolution of the sensor. However, due to their properties, polymeric materials also present a number of challenges.
High homogeneity polymers, which keep their properties even by deformation, are required for low loss waveguides. They must allow replication of nanostructures, which can be printed on a large-scale polymeric foil. Therefore appropriate nanoimprint stamps will be produced during the development phase.
The fabrication technics will be adjusted to the optimized materials, to achieve high-quality grating structures and thus maximize the sensitivity and the resolution of the sensor.
Discrimination between thermal and mechanical deformation of the optical grating represents another challenge. Both effects lead to a similar variation of the sensor signal. To distinguish between the two effects, special grating designs and overlaid gratings will be developed.
For the fabrication of the required nanoscale structures in combination with microstructuring, sophisticated processing methods are required. Moreover interfaces for the combination with other subprojects will be defined. This approach will allow a construction of a network, combining different sensor types.
-
C02 - Planar integrated sensor arrays
Supervisor: Prof. Reithmeier and Prof. Roth
The goal of this subproject is to develop novel types of integrated optical strain sensor arrays based on flexible thin polymer foils. Two optical concepts will be investigated. They rely on coupling of light through custom made polymer waveguide structures and detection of the variation of the transmitted intensity or wavelength as function of the strain exceeded. The main tasks are the design, the production as well as the comprehensive optical characterization of the sensor arrays. Within the two approaches the elongation of specifically prepared extension zones will be measured.
While the intensity-based design measures the coupling intensity between a set of emitter waveguides and their corresponding detector waveguides, the chromatic sensor will be able to determine elongation from a wavelength-shift that a strain on the sensor induces.
Both sensor approaches utilize multimode integrated waveguides for guiding the light, the dimensions ranging from a diameter of 265µm at the moment down to 100µm and 50µm in following iterations. The desired measurement resolution is 50µm/m (microstrain) with the chromatic type, while the intensity-based design is able to measure displacement, misalignment and tilt. With further progress, the performance of the strain sensors with respect to environmental influences shall be evaluated by automated measurements.
The subproject collaborates with subproject B04 for the fabrication of suitable coupling structures for light sources and detectors. Furthermore, the project will adopt and evaluate different manufacturing processes from the B-subprojects and also different polymers from subproject A01 and S02.
-
C03 - Planar-optical polymer foil spectrometer
Supervisor: Prof. Schade
The central component of an optical measuring system for spectral filtering of individual wavelengths is a spectrometer. However, conventional grating spectrometers cannot easily be integrated in a polymer film with planar optical elements. Thus, another approach is taken in this project, using passive planar optical filter elements. Such filter elements are known from telecommunication applications where these so-called arrayed waveguide gratings (AWG) are typically used as multiplexing or demultiplexing elements. This approach is in principle also suitable for the realization of planar optical micro-spectrometers in polymer films.
Figure 1 shows a schematic drawing of an AWG. For the application as a dispersive element (spectrometer) light is coupled from the input waveguide in the first free propagations area (FPA). This area is used to separate the light from the incoming waveguide into a waveguide array; in Fig. 1, an array out of 10 waveguides is exemplary drawn. Each of these waveguides has a different optical path length in order to achieve a phase shift between the light beams before entering the second FPA. The AWG is designed in a way that the optical path length equals a multiple m of the chosen central wavelength of λ0 for each of the waveguides. Therefore, the delay between each waveguide and the next will be chosen to be ΔL = mλ0, so that the original wavefront will be reconstructed on the exit side in the second FPA and constructive interference will occur in the center of the exit waveguide plane which is chosen to be located on the so-called Rowland circle. If a different wavelength than the central wavelength λ0 enters the AWG input waveguide, the constructive interference will be located at a different spot on the exit plane, so that a spatial separation of individual wavelengths is realized. If the output waveguides are now positioned at different locations on the Rowland circle, the AWG can be wavelength-selectively read out using photodiodes connected to these output waveguides. As the constructive interference can occur for a variety of wavelengths at certain points, the free spectral range (FSR) of the AWG is defined as a wavelength or frequency spacing between two maxima of the interference pattern: FSR = nΔλ≈Δλ0/m. Where n is the number of wavelength channels and Δλ is the wavelength resolution.
Since the transmission properties of the AWG are determined by the differences between the path lengths of the individual waveguides, it is crucial to minimize the effects of external influences of these properties such as temperature or mechanical stress. In this subproject it will be examined how far a mechanical stabilization is possible with the implementation of ZnO nanowires or nanostructures and whether an athermal behavior can be achieved by means of a suitable design and material adaptation.
Important work packages in subproject C03 are the simulation, design and optimization of the filter elements and the systematic study of wave guide structures in polymer films modified with orderly embedded nanowires. Figure 2 shows a simple system for the separation of the incoming light into two output waveguides and the results of the associated simulation. Following the specifications of the sensor projects, AWGs can be tailored to meet the requirements of number of channels and thus resolution and covered wavelength range.
To systematically investigate material parameters of modified polymer foils, nanostructures are produced with wet chemical methods. The process was optimized regarding the realization of long and thin nanowires and a high yield.
Figure 3 shows a REM picture of produced nanowires. The incorporation into the polymer is done during polymerization. The modified polymers are characterized regarding their thermal expansion and their dispersion of refractive index. Additionally, the homogeneity of incorporation is investigated. Clustering and subsidence are suppressed with a functionalization of the wires in cooperation with project B06. A further possibility is the use of nanostructures; the data are compared to identify the simplest method for stabilization and to ensure athermal operation.
-
C04 - Resonate and interferometric optical sensors
Supervisor: Prof. Zappe and Dr. Willer
The measurement of gaseous media using optical methods is well-established, e.g. in applications such as industrial safety, environmental monitoring or air conditioning. However, usually measurements are taken only at a few distinct points and therefore no information about the spatial distribution of gases and their concentration is achieved. For many applications a spatially-resolved measurement is of greatest interest. First attempts are the setup of fiber optical systems. The objective of this project, i.e. the functionalization of a polymer foil provides however more potential than the mere multiplexing of fiber sensors since a truly two-dimensional structure is available which is functionalized with sensors at strategic important places.
Interferometric and resonant based on integrated optical structures can be used for transducing chemical or biological data into optical signals. In the close vicinity of a waveguide, an evanescent field is present that enables the interaction between substances surrounding the waveguide and the electromagnetic radiation that is guided within the waveguide. The resulting phase shift or shift in resonance frequency can be used as sensitive measure for the determination of trace amounts of a given analyte.
Within the foil, ring resonators and mach-zehnder interferometer will be realized using single mode waveguides. In selected areas, the cladding is removed; therefore, the core is exposed to the surrounding environment. Due to sensibilization of these surface areas with receptor molecules or structures, the target molecules are adsorbed selectively and are able to interact with the evanescent field. The change of effective refractive index as a result of absorption gives rise to a shift in resonance frequency or a change in interference pattern respectively.
Forboth sensor types the use of single mode waveguides is essential. Therefore, the first work packages focused on the simulation and design of appropriate guiding structures within polymers. Inverted ridge waveguides and strip waveguides are investigated.
Figure 1 and 2 show the setup of the sensors and results for the simulations for intensity distribution and transmitted intensity respectively. In cooperation with projects B01, B02 and S01 first waveguides could be produced (refer to figure 3), which are presently characterized to optimize structure and design and to enhance the achieved sensitivity. For both sensor types the functionalization of the surface is essential for a sensitive and most notably selective interaction.
Presently thiophenoligomers are synthezised which were already used at the TU Braunschweig for an “electronic nose” to survey if they show a sufficient change in refractive index upon interaction with the target molecules. Systematic investigations are performed to identify suitable coatings and structures and coating techniques are adapted for the used polymers.
In a later funding period, arrays of sensors are realized which enable simultaneous measurement of several species and a reliable elimination of cross sensitivities.
-
C05 - Whispering gallery resonators for molecular analysis
Supervisor: Prof. Morgner and Dr. Wollweber
The analytics of the life science takes advantage of various optical methods. Nevertheless application areas could be substantially expanded and flexibilized, if a sensor film with integrated analytics could be made available. Today all samples to be analyzed need to fill into specialized sample containers, adapted to the particular analytical method. Advantage of a flexible sensor sheet would be that it could be adjusted to every sample container. This opens up new fields of application for established analytical methods. Moreover sample containers (lab-on-a-chip, capillary systems, but also larger reservoirs) and sensor technology could be geared to each other by the use this integrated approach. If a full integration, including optical excitation source and detection unit, is successful, it will be a cost-saving alternative to established standard devices (e.g. microtiter plates with integrated readout). The field of application of these full integrated sensor foils range from a continuous monitoring of cell cultures in an incubator, where tissue culture flasks, petri dishes and multiwall plates can be equipped directly with sensor films, to high-content screening of smallest amounts of blood and to process monitoring in the food industry.
The goal of this subproject is to transfer selected optical standard analytics used in life science to planar polymer structures, in order to initialize new or expanded field of application, to develop cost-effective and user-friendly integrated systems and to establish the foundation for development of new measurement systems.
As part of the subproject C05 polymer-based sensors should be develop for the life sciences. This sensors are supposed to enable the optical and spectroscopic analytics in fluidic systems. The target of the first conveyor section is to design a sensor, which is able to detect lowest analytic concentrations (final target single-molecule sensitivity). Operating principle of the sensor are Whispering gallery resonances in microspheres. The resonance frequency and the quality factor of these microsphere resonators are very sensitive to molecules, located in the evanescent field of the spheres. The sensor concept shall be implemented, where every stage is designed in a way, that it forms the basis of simpler polymer-based optical sensors already: a planar sample holder for spectrophotometric measurements and a refractive index sensor. By arranging these sensors in two-dimensional optical networks, they should provide high content screening and local resolution.
To prepare the structures, technologies from various other subprojects are used – mainly hot embossing for sample wells and for superficial multi-mode waveguides and also direct writing of waveguides into the medium by means of fs-laser pulses. The development of refractive-index-matched polymers will also support the sensor development in the first founding period. Initial focus lies on the development, simulation and implementation of sensor concepts in the polymer. As long as the necessary production technologies for polymers are not available and for comparative studies, the sensors will produced from glass substrates. External light sources and detectors, developed by the partner projects, will be integrated gradually.
Project Block S
-
S01 - Polymersynthesis
Supervisor: Prof. Rühe
The goal of the service project is to provide materials developed within the TRR for the generation of large area optical foils. The consortium needs these materials in sufficiently large quantities with well-defined properties and within a short time range. We have pooled these goals into a service project, because the synthesis of these materials is time consuming and requires the build-up of a special know-how which would interfere with the research goals of any of the other projects within the TRR.
The polymers and composites are produced in quantities which are sufficient for structuring and processing tests within the consortium. The materials developed within the TRR will transferred into the service project. Other materials will be synthesized as described in the literature. The service project will upscale the synthetic procedures and compounding techniques such that larger foils can be processed. A further topic of the project is the characterization of the materials and the development of quality standards within the TRR. These efforts will lead to a material database from which all research projects can chose suitable polymeric systems for all respective processing strategies.
-
S02 - Hybrid polyme systems
Supervisor: Prof. Müller
During the funding period of 4 years the research will focus on three main topics:
Modification of refractive index: The refractive index of selected polymers will be significantly increased in the wavelength range of 400 to 1100 nm. The research will focus on methacrylate and epoxy based reactive resin systems. After hardening, these systems form polymethacrylates or epoxides. The increase of refractive index will be achieved by solving electron rich aromatic and non-dyeing compounds (e.g. phenanthrene) which are transparent in the visible range up to their maximum solubility. In case of methacrylates the possibility of increasing refractive index by copolymerisation with sidechain modified methacrylate monomer (e.g. benzyl methacrylate) will be also investigated. This monomer has already an electron rich, aromatic phenyl moiety as a side group which will substantially contribute to a higher refractive index. The aim is to increase the refractive index from 1.49 (pure polymethylmethacrylate) up to 1.56 and from 1.55 (epoxy) to 1.65 (all values for a wavelength of 589 nm).
Adjustment of thermo-mechanical properties: Depending on the monomer type a thermoplastic or thermoset is synthesized. Suitable highly transparent epoxides polymerise mainly as thermosets. However increasing glass transition temperatures and thus increasing continuous operating temperatures can also be achieved by multifunctional monomers, like 1,3-Butandiol-dimethacrylate (BDMA), which compensate the plasticizing effect caused by the dopants. The crosslinking is supposed to prevent increasing polymer chain mobility in order to retain or elevate glass transition temperature. At least the plasticizing effect should be eliminated, it would even be better if it will be overcompensated to achieve a huge maximum continuous operating temperature. Shaping within the shaping related subprojects will be done during polymerisation (thermoplastic and reactive molding, respectively). By adding additives or choosing suitable monomers an individual adjustment of the reactive resin viscosity is possible. This is very important for the different shaping or molding processes. Aspired viscosity values will range from 0.05 to 10 Pa s (at room temperature), depending on the material composition. According to that it will be possible to provide reactive resins which will have customized viscosity and modified refractive index values for e.g. ink-jet and offset printing.
Within the first two milestones these new polymer systems will be handed over non- polymerized and with adjusted viscosity (methacrylates, epoxies) to the printing and reaction molding subprojects. In the same way polymerized and modified methacrylates are delivered to the hot-embossing subprojects. For following material properties adjustments a close cooperation with shaping and characterization subprojects is very important. Furthermore an additional refractive index increase and stabilization of continuous operation temperatures is scheduled to enhance the variety of materials and properties.
Development of luminescent hybrid polymers: In the second half of the project new hybrid polymers which luminescence in the visible range will be developed. Organic rare earth complex compounds, e.g. based on europium, emit light in the visible range after optical excitation. Within the project active i.e. emitting hybrid polymers with customized refractive indices and complex compounds will be developed enabling active waveguide components. In a second step material properties will be matched in cooperation with the other shaping subprojects to achieve a waveguide fabrication with the technologies inside the SFB/TRR123-project.
Do you have any questions about the PlanOS project? Feel free to contact us.
Former Speaker

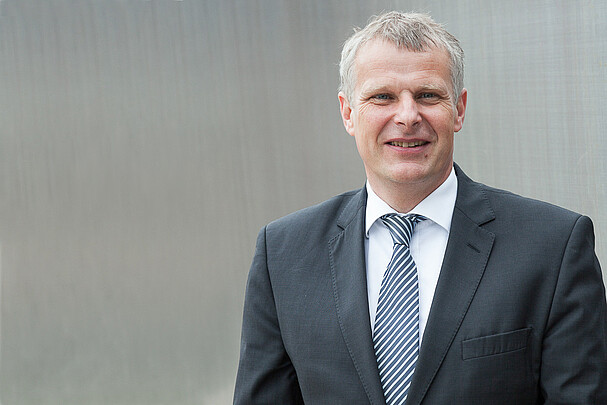
30823 Garbsen

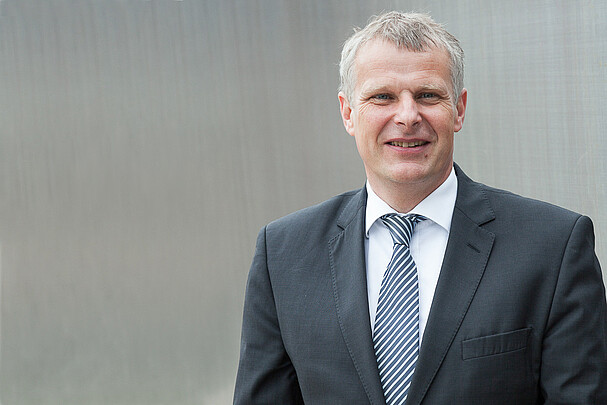
30823 Garbsen
Former Managing Director


30167 Hannover

